
Like many other people I occasionally experience some trouble in keeping Anaximander, Anaximenes and Anaxagoras neatly separated in my mind. I knew that one of the three had been the first to explain wind as "a flowing—river—in the air" but I was not sure which one. Upon looking it up it turned out to have been Anaximander, while Anaximenes had stated that "air is almost bodiless and must be without limit" and also, that "just like our soul, which consists of air, holds our bodies together, so air surrounds all the world." And the third of that trio of similar names, Anaxagoras, had claimed that "air and ether originate from the mass that surrounds them and this is unlimited in extent."
These remarks reveal the first model of the atmosphere, if you want to call it that. It was a very simple model, wherever the soil or the water stopped, the atmosphere began and continued to infinity. Weather was caused by the interaction of air and water, prompted by the sun. And while attempts at understanding the nature of these interactions failed, a number of "signs" were collected which were believed to indicate the next day's weather. Some of them were even valid, if only for the area where they were observed.
A few centuries later Aristotle, when putting all this information together, constructed the second model of the atmospheie. It was a curiously prophetic model, even though it was based on entirely erroneous assumptions. In Aristotle's model, too, the atmosphere extended from the soil to the fiery heavens. But it was no longer uniform as seems to have been silently assumed by the earlier philosophers. It was divided into three layers. The bottom layer in which plants, animals and people lived, was immovable as the earth itself, not counting these local currents which caused the weather. In temperature it was variable. The top layer, touching the heavens, moved with them around the earth and it was also intensely hot. The intermediate layer between the two did not move and was extremely cold—Aristotle knew mountainous regions and the temperature on mountain tops and may have thought that the higher mountains approached this middle layer.
After Aristotle there was little interest in the atmosphere among the "philosophers", the educated classes in our terminology, and what weather knowledge had been gathered slipped back into weather lore, becoming largely superstitious in the process. We must remember that the interest in weather and weather phenomena is directly dependent upon the activities of the people concerned. The nomadic herder shows the lowest interest, if he and his sheep are drenched in a sudden storm, oh well, some god showed displeasure with something or somebody. And it did not matter much. The nomad's interest is confined to seasonal changes, provided that he lives in an area where seasonal changes are pronounced.
The non-nomadic planter of crops is greatly concerned about both, seasonal variations and day-by-day changes. But his concern is strictly localized, he is interested in what the weather will be in his locality tomorrow and the week after. The sailor, on the other hand, is not too deeply concerned about the weather in the place where he is, but would like to know what it will be at his distant port of arrival three weeks from now. But what really interests him is the weather in the intervening area during the intervening time. It is not surprising, therefore, that sailors had the largest amount of weather lore, plain and fancy, and that the sailor's weather lore differed from that of the peasant. It is also not surprising that the first really useful and comprehensive weather notes were systematically taken and collected by a sailor, the English "buccaneer" William Dampier.
That William Dampier was born in 1652, just nine years after Torricelli, a pupil of Galileo Galilei, invented the barometer, thus making the first step beyond Aristotle's collection of "signs" and observations, a time interval of just precisely two thousand years.
But during the time interval a third model of the atmosphere was hinted at by a learned Arab, probably the astronomer al-Biruni who died in 1048. The Arabs had taken Aristotle's works and become acquainted with the Greek conception of the earth as a sphere freely floating in space. On their own they had developed the idea that the stars must be distant suns and al-Farghani—called Alfraganus with a latinized version of his name—had stated quite plainly that even the smallest stars, those of the sixth magnitude, must be still larger than the earth itself. This suggested that our atmosphere might not reach all the way to the sun and to the stars. Since the Arabs also knew a good deal about reflection and refraction, either al-Biruni or one of his contemporaries tried to calculate the height of the atmosphere from the duration of twilight. The result was a figure corresponding to fifty-seven miles or about ninety-two kilometers. We now know that our atmosphere extends higher than that, but beyond a hundred kilometers it no longer has optical properties which could be detected without instruments.
Atmosphere model No. 3 was not very distinct in detail—or, more likeIy, the full description was not preserved—but it was the first with an upper limit. Some five centuries later the newly invented "Torricellian tube"—the name "barometer," was employed for the first time by Boyle—hinted at the same thing. The vacuum which had been so hotly denied by Aristotle did seem possible after all and after climbing a few church steeples a Frenchman by the name of Perier carried the new instrument up the Puy-de-Dome, a mountain in the Auvergne, to prove that there was less air pressure on top of the mountain. Incidentally, the mountain in question falls short of a mile by about six hundred feet. During the following decades there were many such mountain top expeditions, Pascal and Boyle produced theories about gas pressure, the actual existence of a near-vacuum was demonstrated experimentally by Otto von Guericke and the time was ripe for Atmosphere Model No. 4.
Its designer is well known by name, not because of his model of the atmosphere but because he denied Aristotle's notion that comets were atmospheric phenomena.
"Of all the comets in the sky
There's none like Comet Halley
We see it with the naked eye
And periodi-cally.
The first to see it was not he
But yet we call it Halley
The notion that it would return
Was his, origi-nally.
"Dr. Edmond Halley was a man of many accomplishments. He read and used Latin, Greek and Hebrew and when his attention was called to a few presumably valuable Arabic manuscripts he learned and mastered Arabic in order to translate them. On the occasion of an expedition to Newfoundland the Navy lieutenant who had been assigned to him as a navigator, spoke insultingly behind Halley's back about the shortcomings of philosophers in general and of the ship's master in particular. When he repeated the remarks to Halley's face Halley had him confined to his cabin and sailed the ship back to England by himself. There, he prodded Newton into the writing of the "Principia," read all the proofs of the book, made most of the drawings and paid the expenses of publication. But Halley was at his best when it came to putting apparently unrelated facts together and drawing a conclusion from them, as in the case of the comet. In the case of our atmosphere Halley concluded from what facts he had that the atmosphere must have an upper limit and that it consists of three distinct layers. The limit he placed at forty-five miles. The bottom layer extended from sea level to nine miles and was characterized by a steady drop in temperature as one ascended in it. The second layer extended from nine to eighteen miles and was characterized by constant—and very low-temperature throughout. From eighteen to forty-five miles the temperature was supposed to fall off some more, gradually and steadily. Except for the fact that the figures are too small, Halley's model is still valid in most respects.
His definite assertion about the atmosphere's upper limit must have spread widely and rapidly, presumably more through intermediaries than by direct" translation. There is a very interesting passage in a long Latin poem, written in 1767 by one Bernardo Zamagna of Ragiisa and published in Rome one year later. Its title is "Navis Aeria" and it is a description of an imaginary voyage round the world in a balloon—the actual invention of the balloon took place sixteen years later.
"Do not, however," the passage reads, "be lured to ascend too high nor aim by your ambitious flight to surmount the highest peaks of Atlas and Rhodope or the summit of towering Olympus, whose gleaming crest rises above the clouds. There the air has no density and an atmosphere which our race cannot breathe clothes the places far removed from earth; the higher it mounts toward the stars from the land below, the rarer, of course, it becomes and at a very lofty point seems to disappear altogether into nothingness." That was a far cry from the concept of Bishop Francis Godwin who only a century earlier had told that the "ordinary air" reached to the clouds and that the air above the clouds—and all the way to the moon—was so marvelously clear and clean and pure that a traveler had neither the desire nor the need for food and drink.
During the short interval between Zamagna's poem and the actual invention of the balloon, one of the early chemists, Karl Wilhelm Scheele, had discovered that the air was a mixture of two gases and not a compound. Some nine years after Scheele, in 1781, Henry Cavendish published the result of numerous analyses: the air consisted of 79.17 percent—by volume—of nitrogen and 20.83 percent of oxygen. Four years later Cavendish found a strange discrepancy: a small bubble of gas was always left over when he forced all the oxygen and the nitrogen into chemical compounds which were not gaseous themselves. That was actually the discovery of argon, but since argon is chemically inert, Cavendish had no way of attacking or even identifying it. The whole problem was simply forgotten for more than a century, actually until 1894 when Lord Rayleigh noticed the strange fact that atmospheric nitrogen showed a density of 1.2572 while nitrogen gas prepared from chemicals had a density of only 1.2511. Logically there had to be something in atmospheric nitrogen which did not enter into the compounds of nitrogen. As impurities go it was rather massive, more than one percent of the nitrogen and almost one percent of the atmosphere taken as a whole. Lord Rayleigh and Sir William Ramsay found to their surprise that it was a really inert gas, not just comparatively inert like nitrogen or carbondioxide. Remembering the Greek word for "lazy", namely argos, they called it argon. But the chemical investigation of the atmosphere is a later chapter which had to wait for two research tools yet to be invented in Cavendish's day: the spectroscope, which would detect and identify chemically inert substances; and the liquid air machine, which would reduce the atmospheric fluff to palpable liquids that could be handled and fractionated.
However, that early chemical attack on the various "airs"—gases made by Scheele, Cavendish and others had led to the discovery of hydrogen and to the invention of the hydrogen balloon. The hot air balloon had preceded it by a month or so, but the hydrogen balloon could carry more and was more practical all around. The new research tool of the balloon replaced mountain ascents. It was much easier and faster for reaching the same altitude as could be reached by the laborious climb of nearby or not so nearby mountains. In fact it carried much higher than any mountain top in Europe. As early as 1804 Gay-Lussac reached an altitude of 7,016 meters {Note: Since all measurements In meteorological work and high alttiude research are now expressed in metric units I'll stick to the metric system from now on. For those who are not used to it: 1,000 meters ore 1 kilometer, which is the same as 0,281 feet, while 5 miles are almost precisely 8 kilometers}, not quite attaining the 7,400 meters claimed by Robertson and Loest one year earlier. In retrospect one can only be surprised that these men survived their experiences, one should think that they would either have died of anoxia or frozen to death. In fact both came very nearly true. Regarded purely as altitude records these two flights remained unbroken for a long time. I have before me a list of such ascents prepared by a French historian of aviation and according to that list it was not until 1850 that even Gay-Lussac's record was broken. In that year Barral and Bixio ascended to 7,040 meters, but the difference is so small, especially for such an altitude, that it could be more than accounted for by a tiny error of instrument calibration. The record was certainly broken in 1862 by Coxwell and Glaisher whose instruments claimed 8,800 meters. In 1894 Berson succeeded in ascending beyond the 9,000 meter mark—his instruments read 9,150 meters—and the same Berson, flying with Siiring, reached 10,800 meters in 1901. This was probably the highest flight in an open gondola from which the aeronaut returned alive to tell the tale, it required the air-tight gondola of the modern stratosphere balloon to go beyond 11,000 meters with relative safety.
All these high-altitude flights had been strictly scientific in purpose, the gondolas of the balloons were cluttered with barometers and thermometers, containers for taking air samples, magnetic needles with various type's of suspension for obtaining an indication of the earth's magnetic field, et cetera, et cetera. Some of these instruments were already of the type that did not have to be read any more by the aeronaut and they were even then miscalled "self-recording instruments" which is, of course, nonsense since the instrument does not record "itself", but its findings. The findings agreed nicely with each other. The composition of the air did not seem to vary noticeably between sea level and peak altitude. The drop in air pressure agreed with a formula which had been evolved and temperature fell off at a steady rate just as Dr. Halley had predicted. By, say 1890, the rate had been established too. Englishmen said that it was, on the average, one degree Fahrenheit for every three hundred feet of ascent. Frenchmen and Germans said that it was six degrees centigrade per kilometer—both mean the same thing. In addition to the observations from balloon flights a good deal of material from incidental observations of spontaneous phenomena had accumulated.
Scientists living in the Scandinavian countries had tried to measure the height of the aurora by triangulation. It is an undertaking which is more difficult by far than one may think at first glance. But they persevered and amassed figures the lowest of which read around eighty kilometers, the highest—with considerable uncertainty—about five hundred kilometers. Of course nobody was quite sure then just what an aurora was, guesses ranged from electrical discharges to "a reflection of the reflection of sunlight, or even moonlight, on arctic snowfields". But no matter what the guess, the very existence of the aurora proved that there was still something in those altitudes. And then there were the shooting stars. Aristotle had held that they were purely atmospheric phenomena—the annoying similarity of terms like "meterology", "meteoric" and "meteoritic" actually dates back to that assertion—but every once in a while somebody who did not worry about ridicule had wondered whether there might not be an astronomical reason for them. The fight was decided in favor of astronomical origin in the course of the first decades of the nineteenth century, but it was still true that the visible part of the phenomenon took place in the atmosphere. Professors Benzenberg and Brandes of Gottingen decided in 1798 to measure the height of shooting stars.
Now this was a fine and progressive resolution, but it was hard to carry out. While one can see a few shooting stars on almost any night they do not always appear where you happen to look at that instant. And with two observers at some distance from each other—in pre-telephone and even pre-telegraph days—the chances dropped sharply. In short Benzenberg and Brandes were not too successful, but as more and more astronomers began to pay attention to the idea some useful chance observations were obtained. How much chance was involved, even after the introduction of photography, can be seen from the tally of Professor Max Wolf of Heidelberg: the total of the plates he had exposed between 1890 and 1902 amounted to six hundred twenty-five hours and thirty minutes of observation, between them the plates showed nineteen trails of shooting stars. Still, by 1900 astronomers knew that shooting stars usually become visible when they are between one hundred fifty and one hundred eighty kilometers high arid that they disappear between eighty and one hundred kilometers. Of course the big ones will reach all the way to the ground.
And in August, 1883, the island of Krakatoa in the Sunda Sea exploded. That island had three volcanoes, Mount Perboewatan, Mount Danan and Mount Rakata. Mount Perboewatan had started erupting in May 1883, Mount Danan joined it in Tune and Mount Rakata on Sunday, August 26, 1883. After roaring, beginning at 1 :00 p. m. local time, all afternoon and all through the night the volcano blew up at 10:05 a. m. Monday morning, obliterating the two other volcanoes and half of the island. The important point for our theme here is that an estimated cubic mile of steam, carbon dioxide, lava, ashes and soil were thrown into the air. Some of this material formed "noctilucent clouds" which persisted for a long time and could be triangulated accurately and leisurely. Altitude eighty kilometers. The highest other clouds are the so-called mother-of-pearl clouds, forming at an altitude of about twenty kilometers. They are now believed to consist of ice crystals.
During those high altitude balloon flights of the nineteenth century there had been one which ended as a tragedy—as it turned out later it was just this tragedy which was to influence future research more than any successful flight had done. In 1874 two scientists, Sivel and Croce-Spinelli had made a flight which carried them to 7,400 meters. During the following year fhey tried again, the name of their balloon was Zenith and Gaston Tissandier joined them in the gondola. They attained an altitude of 8,840 meters, just about the same as that of Mount Everest. But when the Zenith landed, only Tissandier was still alive, he had just narrowly escaped and is said to have been sickly for the remainder of his life. It was this ill-fated flight of the Zenith which caused meteorologists to do some fundamental thinking. The purpose of such flights was to obtain information about conditions at high altitudes. But that information was not obtained by the aeronauts themselves, it was obtained by instruments. Some of these instruments did not even have to be read but recorded their findings. So why endanger human lives? Why not send up unmanned balloons carrying instruments only?
A man by the name of Brissonet made this suggestion for the first time—in 1879—and others took it up, pointing out that all the instruments one would probably use would still weigh less than a single aeronaut dressed in three fur coats, fur gloves, felt boots, et cetera. Therefore such unmanned balloons would rise higher. And they could also be much smaller and cheaper. In addition to all the men who wrote about this idea and talked about it there were a few which actually went to work, Gustave Hermite and Georges Besancon in France, and Professor Assmann and his assistants in Germany. Hermite and Besancon began by making their own balloons of oiled paper and by buying commercial instruments which they stripped of all excess weight. Later on they said that they had approached the problem as scientists but found that they had to become apprentice mechanics and could not reappear as scientists until after they had grown into full-fledged instrument makers. Their first successful
ballon sonde ascended on March 21, 1893 near Paris and rose to fifteen kilometers. Assmann and his men either dawdled a bit or were too thorough, they were not ready until April 27, 1894. But their balloon rose to twenty-one point eight kilometers. Many more followed vefy quickly, none going much higher for a number of years, but each one contributing more and more information.
And based on this material the next model of the atmosphere was designed in 1898 by the French meteorologist Leon P. Teisserenc de Bort. Both he and Assmann had noticed that Halley's prediction of a constant temperature for a certain height, or rather from a certain height on, held actually true. In his book on meteorology Teisserenc de Bort used and advocated a two-layer atmosphere. The lower layer he proposed to name troposphere—using a Greek word which means "to turn", but used here more in the sense of "to churn"—and all true meteorological phenomena, all "weather", was supposed to take place in that layer. Since vertical currents would be absent in the upper layer the air in it would lie stratified, hence the name
stratosphere was proposed for it. The dividing shell between the two layers was to be called tropopause. The temperature of the stratosphere was established as being -55° C. (-67° F.)
It was already suspected at that time, and was fully confirmed in the years to follow, that the height of the tropopause depended on geographical latitude. There are seasonal variations too, but the figures usually given are: 6 kilometers over the poles, eleven kilometers at Latitude 50°—the latitude of Le Havre, Prague and Kiev in Europe, and of Vancouver Island and Winnipeg on this side of the Atlantic—and eighteen kilometers over the equator.
Most of the two decades that followed after Teisserenc de Bort's publication were devoted to a patient filling in of detail into the picture which he and others had outlined. No major revolution was in sight—it would have needed something approaching omniscience to realize that Marconi's wireless, Lee de Forest's radio tube, Dr. Mach's researches on speed of sound, et cetera, the beginnings of scientific rocket theory and even the mass-production of machine guns all presaged revolutions of the sciences which are concerned with the atmosphere. That Sir William Ramsay in England had followed up Lord Rayleigh's discovery of argon by discovering helium, neon, xenon and krypton was interesting, especially in the case of helium because this gas was known since 1868 spectroscopically as occurring in the sun. On earth it remained hidden until 1894. But this was a filling in of detail, too. That aviation progressed nicely was a help, almost every flight could be combined with research.

For the sake of the aviators a set of tables of atmospheric properties in different altitudes was drawn up, it was useful to meteorologists, too. Although no aviator ever went to twenty kilometers the tables usually ended up with that figure since the material was available. Here's a slightly later version of these tables [above], from NACA Report No. 218, which, it may be remarked, is still valid and useful. The column "speed of sound" is, of course, a later addition, nobody thought that important in 1925 when NACA Report No. 218 was printed for the first time. Now it is, as everybody knows, important for establishing the Mach number, which is defined as the ratio of the true air speed of a plane, bomb, shell or missile to the speed of sound. If we call the airplane speed v and the speed of sound c, the definition looks like this:
M = v/c.
As a glance at the table shows, c varies with altitude and since v is likely to vary with altitude too, the Mach number M cannot be a constant value for a given plane or missile. That is known to most people interested in flying by now, but the vast majority of the people who know it believe that that has to do with the density of the air. The same glance at the table could have shown that this is not the case and in order to demolish the error let's define c in turn. To do it "from the bottom up" would require an extra page, so I'll proceed at once to the final formula which reads
c(2) = kRT
where k is the ratio of the specific heats at constant pressure and constant volume—just remember that the standard for h is 1.4 if you speak of atmospheric air—R is the gas constant and T the absolute temperature. All of which works out to
M(2) = v(2)/c(2) = v(2)/kRT
which demonstrates that the Mach number actually depends on the temperature. We'll find out later at what point that concept has to be demolished too.
Before proceeding to the next Atmosphere Model—I'm beginning to grow hesitant about consecutive numbers at that point—let's get rid of a few necessary figures that should be mentioned. First, the chemical composition of the atmosphere, more or less within the confines of sea level to twenty kilometers. In dry air, at an intermediate latitude, and more than fifty miles from the nearest chemical factory, we have the following:
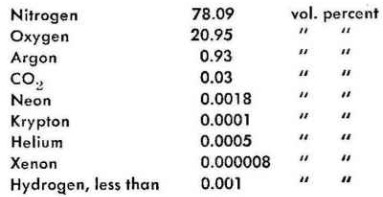
Near the ground you can find a trace of radon, produced by radioactive decay. It never gets high, because it is a very heavy gas to begin with and decays to a lower atomic number before convection currents can carry it up. Conversely, spectroscopic studies have shown that there is slightly more than one part in a million of CH(4)—methane—in a higher layer, tentatively put at eight kilometers. Similarly there are traces of N(2)O in the atmosphere and at a rather high altitude a fairly substantial amount of ozone, about as much as helium, but concentrated in a specific layer. Then there are strict impurities in the air, solid motes, about 100,000 per cubic centimeter in city air but only four hundred or so in the air over the high seas, far from land.
And then there is water vapor, the "humidity" of hot summer days. If our atmosphere were ever completely dry it would weigh 5x10(15) tons—a 5 followed by 15 zeros—including the water vapor it weighs 5.9 x 10(15) tons. Since air of a temperature of less than minus 40°—C or F—has no measurable moisture content any more, all that vapor has to be below nine kilometers. Incidentally, half of all the mass of the atmosphere is below five point six kilometers. And if sea level pressure prevailed throughout the atmosphere it would be 7,995 meters high, the so-called "ballistic atmosphere" which could actually be used for ballistic calculations in the good old days. They neglected the curvature of the earth's surface too and still got usable results.
Most of the things just recited were nicely put on paper at the time of the first World War, with very similar figures. Meteoiologists operated glibly if not always successfully with Highs and Lows, cyclones and anti-cyclones, isobars and isotherms and the force resulting from the earth's rotation. Then came the war and because machine guns had been mass-produced in the meantime the war settled down to fixed front lines and became a long war. The subsequent changer, can all be traced to that one factor. I don't say that they wouldn't have come about anyway in the long run, but the way things actually happened they are all traceable to the long duration of the first World War.
Because of the war there occurred big explosions, not just gun fire and shells but the kind that resulted if somebody succeeded in blowing up somebody else's ammunition dump. What had been noticed occasionally before when a volcano put on a major performance now became a phenomenon for which one could wait: the "zone of silence". An ammunition dump blew up, everybody could hear it within a radius of ten or fifteen kilometers. To those at a distance of twenty kilometers the explosion was too far away to be audible. But at thirty kilometers it was audible again. Apparently something in the air reflected the sound waves to the ground again.
Because of the war communications, still mostly carried by cable, were severed—often literally—and "wireless" was pushed. It could be done and suddenly an engineer realized that he had contact with another station far below the horizon. How could that be since radio waves were supposed to travel in straight lines? Presumably there was also a layer in the air which reflected radio waves. Strangely enough it worked better during the night. And sometimes, or so it seemed, the waves were apparently absorbed instead of reflected.
Because of the fixed frontlines, battleships were robbed of their big rifles which were mounted on railroad carriages and used for long range ground-to-ground work. While engaged in this, the artillerymen were reminded of the fact that the direction of the wind on the ground was not necessarily the same as the direction of the wind at three kilometers. That was in itself not new, but had not been thought of in this correction. The answer was very simple. A small unmanned balloon was released prior to firing and observed telescopically, it told the direction of the wind and gave an indication of its velocity. But what happened if you had a nice solid cloud layer at one kilometer? Officers of the United States Signal Corps had a fine idea. Small transmitters were already available—for airplanes—and they suspended such transmitters from their balloons, hoping to trace their path in spite of low-hanging clouds. It did not work as expected at first, but the idea was filed away for reference "after the war".
And because of the war neutral Norway was in a nasty position. Norway had a large merchant marine and depended for much of its food on its fishing fleet. Both needed weather forecasts and needed them badly. But the weather of the belligerents was a military secret and the Chief Forecaster at Oslo—then still Christiania—had nothing to work with. The result was that he and his colleagues sat down and began to think. Just what did these Highs and Lows, et cetera, et cetera, signify? Well, supposing a cold river of air came down from the North. It was cold and therefore dry. It met a warm river of air from the South which was warm and could, therefore, hold a considerable amount of moisture. But where it met the cold river it was cooled off, could not hold its moisture any more. Then there occurred "precipitation". During the first World War, because of the war, they invented air-mass theory in Norway and because of its wartime origin it still bristles with terms like "stationary front", "advancing warm front" and "breakthrough".
Just before the war, a German, Professor Alfred Wegener, had indulged in some pure theory. Teisserenc de Bort had said, and every day proved it anew, that the troposphere was turbulent. The stratosphere was not, it was stratified. The theory of gas dynamics should enable one to calculate nature an extent of these strata. It was "most obvious" that the gases would separate according to their specific gravity. Near the ground we had the standard mixture, but in the stratosphere nitrogen should become still more preponderant at first, until replaced in turn by hydrogen. Hence you had first the mixed troposphere, then the nitrogen stratosphere, above that the hydrogen sphere and above that the geocorontum sphere. Geocoronium was an unknown element, lighter still than hydrogen, forming the sun's corona. Like helium it might still be discovered in our own atmosphere.
The war delayed the acceptance of that theory for a while so that it did not emerge full strength until 1920-1925. although published originally in 1911. R. H. Goddard coupled a short recounting of that theory with a plea for rockets as research instruments. Svante Arrhenius enlarged upon Wegener's work and published a neat diagram (Figure: "Model 1925").

To tell chronologically what happened after 1925 would only result in confusion, it is now necessary to follow various lines of development without too much attention to their interference and to crossed wires. First let's dispose of the coronium and its assumed twin geocoronium. In 1911, when Wegener advanced the idea, there were still a number of holes in the Periodic Table of the Elements. By 1930 they were virtually all filled in and there simply was no room for the element coronium. But the corona was there and it emitted lines in the spectrum which did not correspond to any known element, even though virtually every element was known. Hence the corona probably consisted of a known element which, because of—to us—highly unusual conditions emitted lines not known. To make a long story short, the unknown coronal lines and a number of others owed their existence to the emissions from "stripped" atoms, atoms which had lost some of their electrons. In the case of the corona a Swedish physicist, B. Edlen, showed in 1940 that the strongest coronal line, the so-called green line, which had been the cause of all the speculation, was emitted by thirteen times ionized iron, iron atoms which had lost thirteen of their normal twenty-six electrons. That ended coronium.
But Wegener's third layer, which he had called the hydrogen sphere, was established without the chemical implication and with a different name by Arthur Edwin Kennelly of Harvard and Oliver Heaviside of England. They proved that there had to be an ionized layer of air above the stratosphere. Logically it came to be called ionosphere and the shell dividing the statosphere from this higher ionosphere was named stralopause. At first radio engineers, in order to honor the two men, called the "reflecting layer" above the stratosphere the Kennelly—Heaviside Layer. But there were some difficulties, it seemed to change altitude constantly, it seemed to disappear at times, in short it did not behave properly. Part of which was due to the fact that experimenters did not always have the wave length they wanted at their disposal. Of course some outright mistakes were made too. And some unforeseen difficulties got in the way.
I know of one reflection experiment with a rather short wave length which sometimes worked nicely and not at all at other times. "Not at all" means just that, no signal was received at the other end and the party concerned telephoned asking in honeyed tones: "Pray, kind sir, why didn't you start your little transmitter on time?", then repeating the sentence in somewhat different language. It turned out that they had picked the wave length which is characterized by maximum absorption in water vapor.
The outcome of it all was that the assumption of just one Kennelly-Heaviside Layer had been a mistake, there are several reflecting layers. The "D-Region" at about fifty kitometers will reflect only very long radio waves—20-500 kilocycles—and lets all others pass. The "E(1)-Region" at about one hundred kilometers reflects the wave lengths used for standard—entertainment- broadcasting, 500 to 1500 kilocycles. It is repeated at about one hundred sixty kilometers as "E(2)-Region". Naturally none of these regions are sharply defined, the altitudes mentioned merely state where maximum ionization normally takes place. The high frequency waves, 1500-30,000 kilocycles, are reflected by the "F(1)- Region" at about two hundred kilometers in daytime, and, if they are short enough to pass F(1), by the "F(2)- Region" at two hundred eighty kilometers at night. During the day F(2) either disappears, or is full of holes, or changes altitude and blends with F(1); it is largely a matter of interpretation and either one of the three explanations, or even all three, may be correct. Occasionally "erratic E" and "erratic F" has been detected traveling vertically, a behavior which might be compared to cirrus clouds suddenly descending quickly and merging with cumulus. Since all these layers are regions of ionization and are ionized by energy entering from space these things are tied up with solar activity. The best one can say at this moment is that we would like to know much more and probably will in 1960.
And that brings us to the current Atmosphere Model of which it is frankly admitted that it isn't as definite by far as we would like it to be. But before its weaknesses can be pointed out, another development has to be discussed, because it led to something very important: modern instrumentation. (
End Of Part One)
Part Two:
Back To:
Disclaimer:The Propagander! includes diverse and controversial materials--such as excerpts from the writings of racists and anti-Semites--so that its readers can learn the nature and extent of hate and anti-Semitic discourse. It is our sincere belief that only the informed citizen can prevail over the ignorance of Racialist "thought." Far from approving these writings, The Propagander! condemns racism in all of its forms and manifestations.
Fair Use Notice: This site--The Propagander!--may contain copyrighted material the use of which has not always been specifically authorized by the copyright owner. We are making such material available in our efforts to advance understanding of historical, political, human rights, economic, democracy, scientific, environmental, and social justice issues, etc. We believe this constitutes a "fair use" of any such copyrighted material as provided for in section 107 of the US Copyright Law. In accordance with Title 17 U.S.C. Section 107, the material on this site is distributed without profit to those who have expressed a prior interest in receiving the included information for research and educational purposes. If you wish to use copyrighted material from this site for purposes of your own that go beyond 'fair use', you must obtain permission from the copyright owner.
Sources: Two superior Yahoo! Groups, pulpscans and magazinearchive, are the original sources for the scans on this page. These Groups have a number of members who generously scan and post rare and scarce magazine and pulp scans for no personal gain. They do so for love of the material and to advance appreciation for these valuable resources. These Groups are accepting new members; join now!
